Dr. Vladimír Tkáč1
Talk: "Low-temperature heat capacity of Layered Double Hydroxides"
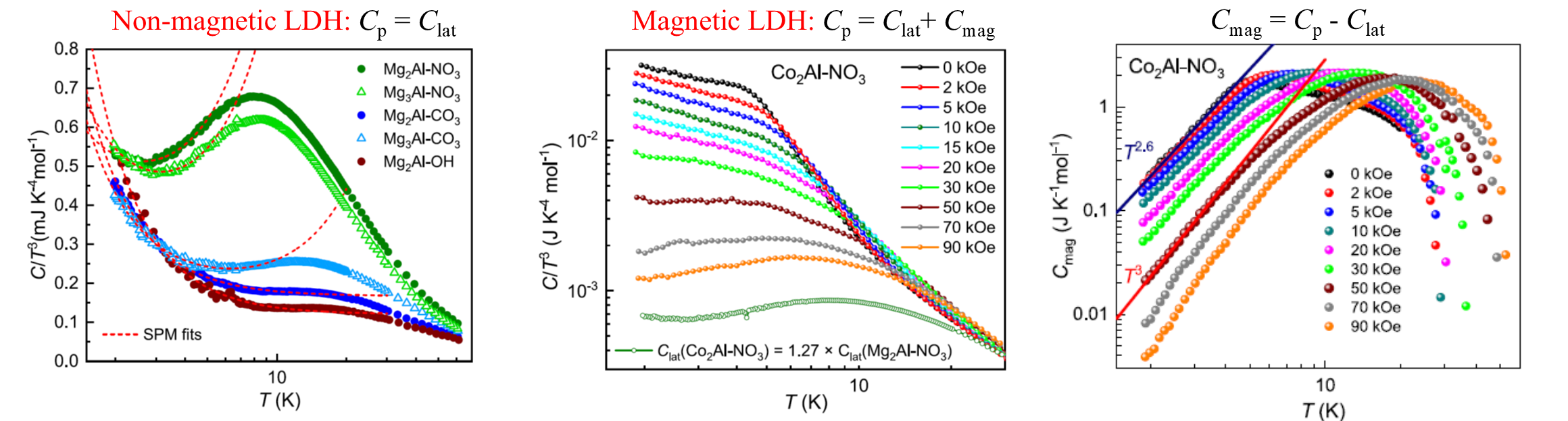
Heat capacity of cobalt-aluminium and magnesium-aluminium layered double hydroxides (LDH) with the divalent-to-trivalent cations ratio (n) of 2 and 3 and intercalated with NO3-, CO32- and OH- was measured as a function of temperature between 2 and 100 K. The total heat capacity, C(T), was found to demonstrate a Schottky-like anomaly [1] observed as a broad local maximum in the temperature dependence below 10 K. Application of the magnetic field results in a widening of the anomaly range and a shift to higher temperatures [1]. Magnetic contribution to the heat capacity (Cmag) of ConAl LDH was extracted from comparison of the C(T) dependences with the respective reduced dependences of their non-magnetic counterparts, MgnAl LDH [2]. In a zero magnetic field, the low-temperature Cmag of Co2Al LDH is proportional to T2.6, while for Co3Al LDH, the low-temperature Cmag proportional to T2 is observed. An application of an external magnetic field was revealed to converge these dependences to the low-temperature Cmag proportional to T3 in compounds with both n=2 and 3. However, the conversion in Co2Al LDH occurs at lower external magnetic field values (50 kOe) than that in Co3Al LDH (90 kOe). The temperature-dependent ac magnetic susceptibility analysis found that Co2+ - Al3+ LDH exhibit a magnetic cluster spin glass behavior regardless of their basal spacing value and the Co/Al ratio [3]. It was revealed from the C(T) behavior of the non-magnetic LDH at the lowest measured temperatures that they possess the features typical for both a 2D solid and a structural glass. The reduced heat capacity C(T)/T3 of MgnAl LDH intercalated with nitrate demonstrates a so-called boson peak, which is a manifestation of a glassy state. Such a low-temperature structural glassy state in LDH is associated with the disorder of the intercalated species. The observed temperature-dependent heat capacity of non-magnetic LDH was fitted using the modified Soft Potential Model (SPM) [2].
- [1] Yu.G. Pashkevich, E.L. Fertman, A.V. Fedorchenko, D.E.L. Vieira, C.S. Neves, R.Yu. Babkin, A.А. Lyogenkaya, R. Tarasenko, V. Tkáč, E. Čižmár, A. Feher, A.N. Salak, Appl. Clay Sci. 233 (2023) 106843.
- [2] V. Tkáč, Yu.G. Pashkevich, E.L. Fertman, R. Tarasenko, E. Čižmár, A. Feher, A.V. Fedorchenko, C.S. Neves, D.E.L. Vieira, A.N. Salak, Appl. Clay Sci. 266 (2025) 107695.
- [3] E.L. Fertman, A.V. Fedorchenko, E. Čižmár, Yu.G. Pashkevich, M. Holub, V. Tkáč, R. Tarasenko, A. Feher, C.S. Neves, D.E.L. Vieira, A.N. Salak, Chem. Phys. Lett. 859 (2025) 141745.
Acknowledgements: The work was supported by the project APVV-22-0172.